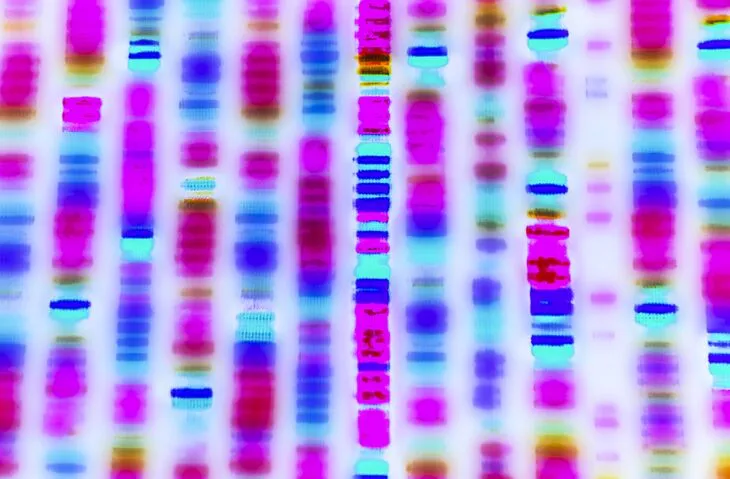
Astrix Partner Blog – What is Next Generation Sequencing, and How Does it Challenge Lab Informatics?
Astrix works with thought leaders throughout the Life Science industry. This includes a variety of technology partners with whom we collaborate on projects and industry thought leadership. As part of this we feature high quality content from our partners to help our customers and website visitors learn about new technologies, process and strategy that may help move their R&D operations through a digital transformation! This partner blog is featured by Sapio Sciences
Next-generation sequencing (NGS) has matured significantly since the first massively parallel signature sequencing was introduced in 2000. For context, the Human Genome Project provided its first “rough draft” that same year. Today, NGS is powering gene-based research, with applications in personalized medicine, gene and cell therapy, metagenomics, epigenetics, and more.
But what is NGS, and how does its implementation challenge lab informatics? Here’s Sapio’s take on the tech, covering the following topics:
- What is NGS?
- How are labs using NGS?
- What is the typical NGS workflow?
- What are the most popular sequencers used for NGS?
- How does NGS challenge lab informatics?
- What’s next for NGS?
- Discover Sapio’s out-of-the-box NGS platform
What is NGS?
The first methods for sequencing long DNA were developed independently in 1977 by Frederick Sanger in the UK and Walter Gilbert in the U.S. Both were awarded the Nobel Prize in 1980, and the techniques they developed are now known as Sanger sequencing. Sanger sequencing is a fast and cost-effective way to sequence low numbers of targets and samples. In fact, because of its accuracy and high data quality, labs today often use Sanger sequencing to confirm NGS results.
Spurred by the need to understand more about the role of genes in protein expression, phenotypic traits, and disease states increased, scientists sought ways to sequence more DNA faster. The result is NGS: massively parallel techniques that enable scientists to sequence millions of DNA fragments simultaneously in a single run or hundreds to thousands of genes.
Compared to Sanger sequencing, NGS supports higher throughput, more rapid processing, smaller sample sizes, and more accurate and deep analysis of massive volumes of data. It’s also made sequencing more cost-effective. For instance, in 2007 when Sanger sequencing was still the dominant sequencing technology, the cost to sequence a whole human genome was $10 million. Today, using NGS, whole genome sequencing costs less than $1,000, and the goal now is to lower the price of sequencing a whole genome to $100.
How are labs using NGS?
With NGS, scientists can powerfully interrogate genetic data, revolutionizing research and clinical studies in the pharmaceutical, biotechnology, and life science industries.
Labs use NGS to study biological processes, disease mechanisms, and evolutionary relationships in a research setting. The following disciplines depend on and likely wouldn’t exist without NGS:
- Proteomics, the expression of proteins in a cell
- Transcriptomics, the analysis of the RNA sequences transcribed in a cell
- Metagenomics, the study of the genetic material recovered from bulk samples such as water or soil
- Epigenetics, the study of changes in cell function caused by factors other than genetics
Clinically, NGS has enabled routine and cost-effective whole genome sequencing, powering a wide variety of clinical applications such as:
- Identifying genetic disorders and disease-causing genetic variations in a person’s genome, either to inform treatment or determine an individual’s risk profile for inherited diseases
- Sequencing tumors or other patient samples, such as blood, to identify mutations that may respond to targeted treatments
- Monitoring disease progression and assessing how patients respond to treatment, including using pharmacogenomics to adjust dosing individually for maximal safety and efficacy
- Screening fetal and newborn genomes for genetic disorders, enabling physicians and families to intervene and treat diseases earlier
Clinical NGS focuses on translating genomic information into actionable patient care and management insights. This requires labs to enforce rigorous quality control (QC), adhere to regulatory standards, and provide secure, user-friendly portals through which physicians can interpret the data to make patient decisions. These requirements present informatics challenges to labs seeking to implement and manage clinical NGS workflows.
What is the typical NGS workflow?
At its most basic, the NGS workflow comprises three primary steps: library preparation, sequencing, and data analysis. Most instrumentation vendors provide library preparation kits that build a library of fragments for sequencing. Therefore, library preparation encompasses several embedded workflow steps that labs must manage, including:
- Handling sequencing requests
- Managing samples from request to reporting results
- Tracking the use of reagents, library kits, and other consumables during the workflow
- Conducting appropriate QC, especially in clinical applications
- Interfacing with instrumentation and automation at all stages to speed nucleic acid extraction, library preparation, QC, pooling, and sequencing
What are the most popular sequencing instruments used for NGS?
The most widely used instruments for next-generation sequencing are:
- Illumina sequencers. These platforms, which come in benchtop and production formats, employ sequencing-by-synthesis (SBS) technology, in which DNA fragments are amplified, attached to a flow cell, and sequenced using reversible terminators. Illumina sequencers use fluorescence to read bases in the nucleotide sequence. Illumina sequencers account for 90% of the world’s sequencing data.
- IonTorrent sequencers. Like Illumina’s platforms, IonTorrent sequencers also use SBS technology. Instead of employing fluorescence to read sequences, IonTorrent systems generate nucleotide sequences using a semiconductor chip that measures changes in pH caused by the release of hydrogen ions during DNA polymerization.
- Pacific Biosciences (PacBio) sequencers. Illumina and IonTorrent sequencers use second-generation sequencing technology, which requires PCR amplification of template DNA. PacBio utilizes single-molecule sequencing (SMS), a third-generation sequencing technology. SMS requires less starting material and generates fewer errors. PacBio platforms are particularly good at generating long reads, enabling better characterization of complex genomic regions, structural variations, and epigenetic modifications.
- Oxford Nanopore sequencers. These sequencers employ fourth-generation sequencing technology, which performs real-time sequencing in situ without amplification or synthesis. DNA or RNA strands pass through nanopores, and the sequencer measures changes in electrical current to identify bases. Nanopore sequencing has made whole genome sequencing faster and less expensive and can be used in conjunction with second-generation sequencing technology to identify the order of nucleotides in fixed cells and tissues.
How does NGS challenge lab informatics?
NGS workflows continue to evolve with the development of new sequencing technology. Labs seeking to support NGS workflows face the following lab informatics challenges:
- Tracking up to thousands of variants and metadata per sequencing run. Informatics must support scientists in receiving requests and samples, queuing them up for sequencing, and processing them through results. It must also provide real-time ways to report on sample status through the workflow to keep requestors informed.
- Integrating with instruments and other systems is needed to support sequencing. This includes sequencers, reagent and sample management systems, quantification systems, and primary, secondary, and tertiary analysis tools.
- Handling errors and quality control. Software can streamline workflows by automating KPIs and providing easy ways to reprocess samples that fail QC.
- Managing data privacy and security. This is especially important in clinical labs sequencing data from patients. Software can strip personally identifiable information from samples during processing and reassociate it once sequencing is complete to support decision-making. It can also set permissions so that scientists have access to the right information relevant to their work.
- Supporting collaboration and data sharing. Software can struggle to support specific tasks in a complex workflow and provide a summary of the entire work queue and KPIs achieved over time. Scientists also need easy ways to share data with colleagues to gain insights and feedback on experiments.
- Scaling and adapting as workflows change. Software can lock in labs to a particular way of working or break when new functionality is inevitably introduced. To keep pace with NGS’ evolution, labs need out-of-the-box NGS, templated workflows built into a no-code/low-code, easily configurable platform that will enable them to adapt at the speed of science.
What’s Next for NGS?
Sequencing technology continues to advance, with costs decreasing faster than processing speeds predicted by Moore’s Law. To stay on the forefront of this technology and deploy it effectively and efficiently, labs need software that can handle end-to-end NGS workflows and enforce standardized procedures for quality control, data handling, and analysis.
About Astrix
Astrix is the unrivaled market-leader in creating & delivering innovative strategies, solutions, and people to the life science community. Through world class people, process, and technology, Astrix works with clients to fundamentally improve business & scientific outcomes and the quality of life everywhere. Founded by scientists to solve the unique challenges of the life science community, Astrix offers a growing array of strategic, technical, and staffing services designed to deliver value to clients across
their organizations.
Case Study: LabWare Centralized Data Review for a Global Biopharmaceutical Company
Overview A global biopharmaceutical company specializing in discovery, development,... LEARN MOREWhite Paper: Managing Data Integrity in FDA-Regulated labs.
New White Paper LEARN MORELET´S GET STARTED
Contact us today and let’s begin working on a solution for your most complex strategy, technology and staffing challenges.
CONTACT US